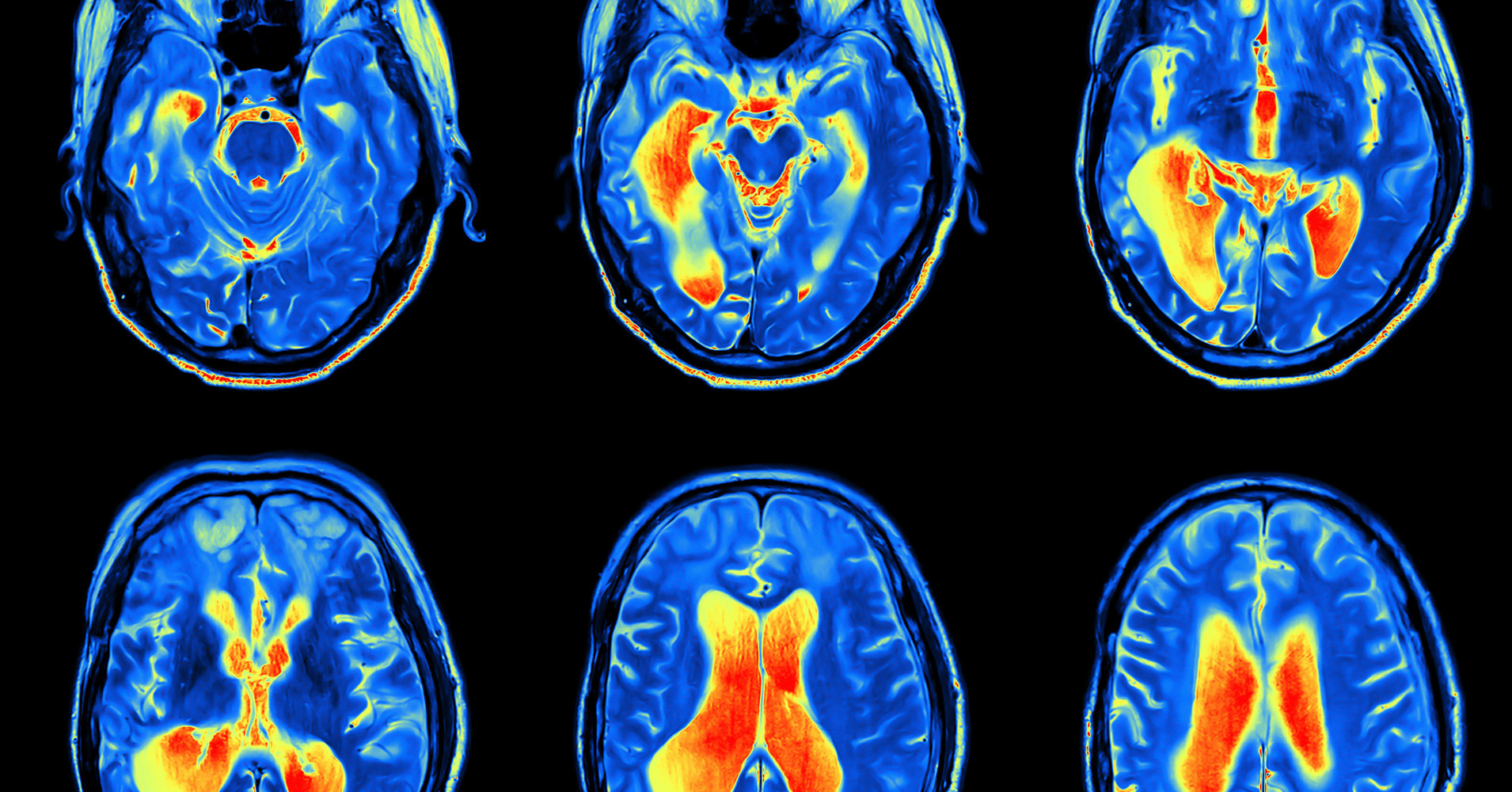
In hospitals and on television, scenes of patients undergoing radiation therapy for cancer or receiving PET scans for diagnosis are not uncommon. These medical procedures are part of the intricate world of nuclear medicine, a field that utilizes radioactive substances to both image the body and treat diseases. Nuclear medicine, unlike other diagnostic methods, looks not only at the anatomy but also at the physiology, unraveling the functioning of organs and tissues to establish accurate diagnoses and treatment plans.
Imaging in Nuclear Medicine:
The human body, opaque and often resistant to direct observation, has historically compelled physicians to resort to invasive techniques like exploratory surgery. However, the contemporary medical landscape is adorned with a plethora of non-invasive technologies, including X-rays, MRI scanners, CAT scans, and ultrasound. Each of these techniques carries its own set of advantages and limitations, catering to diverse conditions and anatomical regions.
Nuclear medicine imaging emerges as a powerful ally in the quest to explore the human body’s intricacies. These techniques amalgamate computers, detectors, and radioactive substances to delve deep into the physiological and anatomical dimensions. Prominent among these techniques are Positron Emission Tomography (PET), Single Photon Emission Computed Tomography (SPECT), cardiovascular imaging, and bone scanning, each leveraging the distinctive properties of radioactive elements.
Video: What is nuclear medicine?
Detecting Deeper Realities:
The versatility of nuclear medicine imaging is evident in its ability to detect a range of conditions, from tumors and aneurysms to irregular blood flow and organ malfunctions. The choice of a specific imaging test, or a combination thereof, is tailored to the patient’s symptoms and the nature of the disease under consideration.
Positron Emission Tomography (PET):
PET, a flagship technique in nuclear medicine, generates intricate body images by capturing the radiation emitted from injected radioactive substances. These substances, tagged with short-lived radioactive atoms like Carbon-11 and Fluorine-18, emit gamma rays detected by PET. The patient, injected with the radioactive substance, moves through a circular gamma ray detector array, producing thin slice images assembled into a three-dimensional representation of the body. PET provides invaluable insights into blood flow and various biochemical functions, offering images of glucose metabolism in the brain and dynamic changes in different areas of the body.
SPECT, Cardiovascular Imaging, and Bone Scanning:
SPECT, akin to PET, employs radioactive substances like Xenon-133 and Technetium-99. Distinctly, SPECT emits single gamma rays, providing information about blood flow and substance distribution. Although SPECT images may lack the sensitivity and detail of PET, its cost-effectiveness and accessibility make it a viable alternative. Cardiovascular imaging, a subset of nuclear medicine, traces blood flow through the heart and vessels. Techniques like stress thallium tests, involving radioactive thallium compounds and gamma ray imaging, aid in detecting blocked arteries or arterioles in the heart. Bone scanning, another critical application, detects radiation from a radioactive substance, particularly useful in identifying tumors with heightened metabolic activity.
Treatment in Nuclear Medicine:
Contrary to popular belief, radioactive substances used in nuclear medicine imaging tests do not harm the body. The radioisotopes employed decay rapidly, with lower radiation levels than traditional X-rays or CT scans, and are naturally eliminated. Nevertheless, ionizing radiation, comprising alpha, beta, gamma, and X-rays, has profound effects on certain cells, especially those that multiply rapidly.
Navigating the Intricacies of Radiation:
Understanding the impact of ionizing radiation on cells is pivotal in comprehending nuclear medicine’s dual role in imaging and treatment. Cells, endowed with mechanisms to repair damaged DNA and, if necessary, to self-destruct, respond differentially based on their multiplication rates. Rapidly multiplying cells, characteristic of many cancers, are prime targets for radiation therapy. This therapy involves strategically placing radioactive wires or vials around tumors, or for deep-seated tumors, focusing high-intensity X-rays on the affected area.
The caveat of such treatment lies in the collateral damage inflicted on normal cells with similar multiplication rates, resulting in side effects like hair loss and nausea. Cells lining the stomach and intestines, skin cells, blood cells, and hair cells are all vulnerable to the effects of radiation, making cancer treatment a delicate balance between targeting malignant cells and preserving the healthy ones.
Harnessing Radioactive Tracers:
Beyond imaging and treatment, nuclear materials are instrumental in creating radioactive tracers for injection into the bloodstream. One variant of these tracers flows in the blood, allowing for the visualization of blood vessel structures and the detection of abnormalities such as clots. Additionally, certain organs concentrate specific chemicals, a trait exploited in diagnostic procedures. For instance, the thyroid gland concentrates iodine, enabling the detection of thyroid tumors by injecting radioactive iodine. Similarly, cancerous tumors concentrate phosphates, detected by injecting the radioactive phosphorus-32 isotope.
Conclusion:
In the realm of medical marvels, nuclear medicine stands as a testament to human ingenuity and scientific progress. The ability to peer into the body’s deepest recesses and to target diseases with precision underscores the transformative impact of this field. As technology advances, nuclear medicine promises even greater insights, making significant strides in the ongoing pursuit of comprehensive healthcare.